Whether in the presence or absence of adequate soil moisture in the soil profile, heat stress can be an important risk and/or limitation to plant growth and development. While some crops are more susceptible to heat stress during critical growth stages [e.g., pollination for corn and R3 (flowering) stage for soybean], both corn and soybean are also susceptible to extreme heat (and water) stress during early vegetative stages.
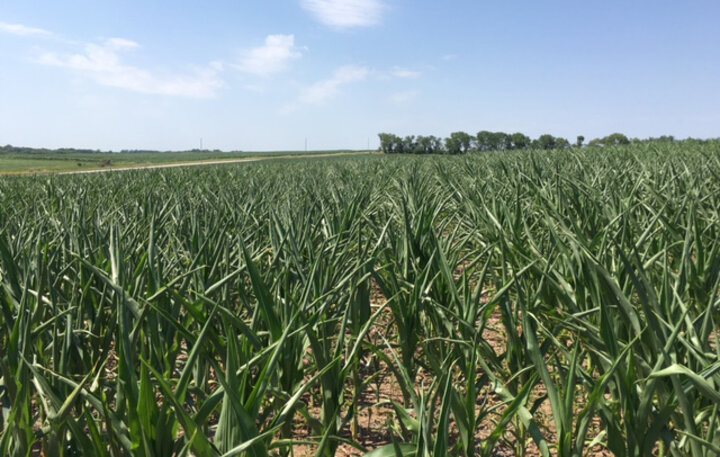
Extreme heat stress can reduce plant photosynthetic and transpiration efficiencies and negatively impact plant root development, which collectively can negatively impact yield. The decline in the photosynthetic rate under both heat and water stresses are frequently attributed to
- lowered internal plant CO2,
- inhibition of photosynthetic enzymes (e.g., Rubisco) and
- synthesis of ATP (adenosine triphosphate), which produces chemical energy that is needed for regulating plant biochemical reactions.
The coupled effect of both heat and water stress on yield of many crops, including corn and soybean, is much stronger than the effect(s) of individual stress alone. Heat stress, in general, is defined as the rise in air temperature beyond a threshold level for a period sufficient to cause permanent damage to plant growth and development. Heat stress is a complex function of intensity, duration, and the rate of the increase in air temperature. In addition, the effect of an increase in soil temperature (as a result of increase in air temperature) may be even stronger when accompanied by a decline in soil water content. Therefore, during extreme heat periods soil water content must be kept at an adequate level 1) to provide for plant uptake and 2) to minimize the impact of higher soil temperature caused by higher air temperature.
When soil water distribution in the plant root zone is heterogeneous, which is the case in most Nebraska soils, plant roots can compensate for drier soil layers by taking more water from wetter layers. However, when there is a heat wave and soil temperatures increase, plant roots are less able to compensate for varying levels of soil moisture within the soil profile. When soil temperature rises above an optimum threshold, plant water and nutrient uptake can be impeded, causing damage to plant components. Extreme air temperature coupled with extreme soil temperature can cause varying degrees of damage to different parts of plants.
My research shows that extreme air and soil temperature can alter the water transport rate from the soil into the root and plant system, which can reduce plant transpiration rate where plant transpiration cannot keep pace with high atmospheric evaporative demand (due to high air temperature). Extreme heat stress (even in the presence of adequate soil moisture) can cause a reduction in plant stomatal conductance, which reduces plant transpiration rate, causing reductions in plant productivity and yield. If extreme heat stress is coupled with dry wind over the plant canopies, the magnitude of stomatal closure and the reduction in rate of transpiration is greater for corn and soybean. If the heat stress is coupled with water stress, this would cause an increase in root clumping, which will decrease the efficiency of plant water uptake. If an increase in root growth can be observed at the onset of water stress, the continuing water stress — especially in the presence of heat stress — will reduce overall root growth.
In general, shallow-rooted crops are less drought tolerant than deep-rooted species such as alfalfa or maize. Under water stress, some plants develop short suberized roots, as the top soil becomes dry. This can help plants survive a drought by reducing water loss from plant roots, but it will impact plant growth, development, and productivity. Therefore, it is crucial to have adequate soil moisture in the soil profile during heat wave periods.
While numerous research studies have focused on heat and/or water stress impact(s) on plant growth, development and yield during reproductive stages (i.e., tasseling, silking, grain formation stages for corn; R3 stage for soybean, etc.), heat stress for a prolonged period in early stages of vegetative growth can also substantially influence crop growth, development and yield. (A heat wave is described here as air temperature equal to or greater than 90oF for 7-10 days or longer.) This effect is especially true if the heat stress is coupled with soil water deficit and/or an increase in soil temperature.
While plant root growth under “early stages of heat and water stress” is generally enhanced for better access to water and dehydration avoidance, prolonged heat and water stress results in root shrinkage, anatomical deformations, and weak root-soil contact that limits water and ion supply. In legume crops, the stresses decrease nodule size and weight and nitrogenase activity.
Is there anything that can be done to mitigate extreme heat stress on plants?
While options are limited for mitigating the negative impact(s) of extreme heat stress on crops, there are several practical options, especially in irrigated settings.
- Monitoring soil moisture during heat wave periods is critical. Maintaining adequate moisture in the soil profile is crucial to reducing the impact of heat stress on crops. Tools and procedures for effective irrigation management are available on the Nebraska Agricultural Water Management Network (NAWMN; Irmak, 2005; Irmak et al., 2010) website.
- While we use advanced instruments in our research programs to decisively distinguish heat stress from water stress (and nitrogen stress), a quick, practical way to check for heat stress is to evaluate the leaf and canopy structure during the early morning hours when air temperature is low. If the leaves are curled and soil moisture in the soil profile is adequate, the symptom is most likely due to heat stress. Curled leaves can also reduce the light/radiation interception and this, in turn, can negatively impact plant water and nutrient uptake and transpiration rates. Another practical way of checking for turgidity of the plant leaves and stems is to break the leaf and/or stem and check for the status of the plant water (sap) in early morning hours and repeat this again during solar noon hours (around 3 p.m.) when air temperature is usually at its maximum.
- My research shows that for center pivot-irrigated crops, even if the soil profile has adequate soil moisture, making a run with the pivot and applying 0.25-0.40 inch of water can be very beneficial to reduce the sensible heat (heat that is used to heat the soil-plant environment) during a heat wave. The heat that exists in the environment will be used to warm up (and evaporate) this additional water and this would, in turn, reduce the soil-plant canopy temperature due to evaporative cooling. The interval for this pivot run with a small amount of water can be every three to five days, depending on the irrigation well, center pivot capacity, and duration of heat wave.
- The process of making a quick run with the center pivot and applying 0.25-0.40 inch of water is not only beneficial during early growth stages, but it can also be effective and beneficial to cool down the plant canopy and help enhance plant metabolic functions/activities during heat waves in reproductive stages as well.
- Similar options for gravity-irrigated and subsurface drip-irrigated crops are limited. However, my research shows that subsurface drip-irrigated fields are naturally cooler than center pivot-irrigated fields due to increased transpiration rates in the subsurface drip-irrigated field that result in greater evaporative cooling. More energy (heat) is used in the subsurface drip-irrigated field than in the center pivot field and this reduces the amount of heat in the environment.
- Adding this additional small amount of water can be especially beneficial if the crops are in very early vegetative stages where their roots are not as developed. Applying a small amount of water should not prevent plants from developing roots into deeper soil layers because plant roots penetrate deeper into the soil profile for many reasons in addition to searching for soil moisture. Other reasons include:
- developing a stronger plant structure,
- searching and uptaking soil nutrients and micronutrients, and
- maintaining adequate root-shoot ratio, etc.
- It's more critical in coarse-textured soils (e.g., sandy, sandy-loam, and similar soils) than in more fine-textured soils (e.g., silt-loam, silty-clay loam, and similar soils) to keep the upper soil layer in adequate soil moisture in early growth and development stages.
- My research showed that in reduced or no-till corn and soybean fields, soil temperatures in the surface soil layers can be up to 8-10oF lower than in disk-tilled fields. Reducing soil temperature during heat waves can greatly help to mitigate the impact of heat stress on plants. Additional residue on the soil surface in reduced and no-till fields can minimize the radiation (heat) interception on the soil surface and alter the heat exchange between the soil and surrounding microclimate. This reduces soil temperature. Reducing soil temperature is beneficial for enhancing root growth and water and nutrient uptake during heat wave periods.
- Soil fertility can also impact the degree to which heat and water stress affect plants. Plant stomatal conductance and the transpiration rate under water stress conditions were observed to be lower under good compared to low fertility conditions. Also, under heat stress soil fertility improved by applying macronutrients such as potassium and calcium and micronutrients such as boron, manganese, and selenium. These applications modified stomatal function and activated physiological and metabolic processes that helped in upholding high tissue water potential and increasing heat stress tolerance.
References
Beudez N. and Doussan C., 2013. Influence of three root spatial arrangement on soil water flow and uptake. Results from an explicit and an equivalent, upscaled, model. Procedia Environ. Sci., 19(0), 37-46.
Gliñski J. and Lipiec J., 1990. Soil Physical Conditions and Plant Roots. CRC Press, Boca Raton, FL, USA.
Irmak, S., D.Z. Haman, and R. Bastug. 2000. Determination of crop water stress index for irrigation timing and yield estimation of corn. Agronomy Journal 92(6):1221-1227.
Irmak, S., and D. Mutiibwa. 2009. On the dynamics of stomatal resistance: Relationships between stomatal behavior and micrometeorological variables and performance of Jarvis-type parameterization. Transactions of the ASABE 52(6): 1923-1939.
Irmak, S., and D. Mutiibwa. 2009. On the dynamics of evaporative losses from Penman-Monteith with fixed and variable canopy resistance during partial and complete maize canopy. Transactions of the ASABE 52(4):1139-1153.
Irmak, S., and D. Mutiibwa. 2010. On the dynamics of canopy resistance: Generalized-linear estimation and its relationships with primary micrometeorological variables. Water Resources Research 46:1-20, W08526, doi: 10.1029/2009WR008484.
Lipiec, J., C. Doussan, A. Nosalewicz and K. Kondracka. 2013. Effect of drought and heat stresses on plant growth and yield: A review. Int. Agrophys. 27, 463-477 doi: 10.2478/intag-2013-0017.
Muller B., Pantin F., Génard M., Turc O., Freixes S., Piques M., and Gibon Y., 2011. Water deficits uncouple growth from photosynthesis, increase C content, and modify the relationships between C and growth in sink organs. J. Exp. Bot., 62, 1715-1729.
Payero, J.O. and S. Irmak. 2006. Variable upper and lower crop water stress index (CWSI) baselines for corn and soybean. Irrigation Science 25:21-32.
Sekhon H.S., Singh G., Sharma P., and Bains T.S., 2010. Water Use Efficiency Under Stress Environments In: Climate Change and Management of Cool Season Grain Legume Crops (Eds S.S. Yadav, D.L. Mc Neil, R. Redden, and S.A. Patil). Springer Press, Dordrecht-Heidelberg-London-New York.
Simoes-Araujo J.L., Rumjanek N.G., and Margis-Pinheiro M., 2003. Small heat shock proteins genes are differentially expressed in distinct varieties of common bean. Braz. J. Plant Physiol., 15, 33-41.
Tardieu F., Bruckler L., and Lafolie F., 1992. Root clumping may affect the root water potential and the resistance to soil-root water transport. Plant Soil, 140, 291-301.
Wahid A., Gelani S., Ashraf M., and Foolad M.R., 2007. Heat tolerance in plants: an overview. Environ. Exp. Bot., 61, 199-223.
Wang Z., Xiong Y., and Wang M., 2000. Study on expert system of irrigation forecast and decision making for water saving. Institute of Agricultural Soil Water Engineering of North West Science and Technology University of Agriculture Forestry, Yangling, Shaanxi.
Waraich E.A., Ahmad R., Halim A., and Aziz T., 2012. Alleviation of temperature stress by nutrient management in crop plants: a review. J. Soil Sci. Plant Nutrition, 12(2), 221-244.